In spite of their pivotal role in observational cosmology, type Ia Supernovae (SNe Ia) are still misterious objects. According to the observational evidence, they are produced by the thermonuclear disruption of Carbon-Oxygen (CO) White Dwarfs (WDs) accreting matter from their companions in binary systems. If the WD total mass increases up to the Chandrasekhar mass limit (MCh), the resulting strong compression determines in the inner zones the physical conditions suitable for C-ignition in highly degenerate physical conditions, so that the explosion occurs. However, no clear consensus there exists so far concerning the typology of the stellar companion as well as the evolutionary path driving to the explosion. Up to now, two different scenarios for the progenitors have been proposed, the so called Single Degenerate (SD) scenario, the donor star being a normal star with a H-rich envelope, and the Double Degenerate (DD) scenario, the donor being a second CO WD with total mass of the binary system equal to or larger than MCh. It is worth noticing that the definition of an evolutionary scenario defining both the evolution up to the explosive C-ignition as well as the physical properties of the explosion itself is mandatory to fully understand the reliability of SNe Ia as standardized candles on cosmological distances.
During the ’90 we started at the INAF-Osservatorio Astronomico di Teramo a longstanding project devoted to systematically investigated the physical properties of binary systems with donor stars of different type in order to individuate the right SNe Ia progenitor system.
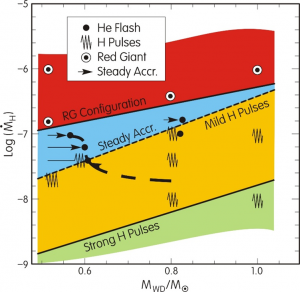
In a series of work [1,2,3,4], we explore the possible final outcomes of CO WDs accreting H-rich matter. Our results, summarized in Figure 1, clearly suggested that it is almost impossible that by accreting H-rich matter the CO WD could attain MCh. In fact, for low value of the mass transfer rate the accreting WD undergoes recurrent dynamical nova-like flashes which eject all the material previously accreted and in some cases erode also the original CO WD. On the other hand, for intermediate and high values of the accretion rate, the accreted hydrogen is nuclearly processed either quiescently or via recurrent non-dynamical flashes thus determining the accumulation of a He-rich layer. However, due to the values of the rate at which such a zone is effectively piled-up, He-burning is ignited via a strong, non-dynamical flash, which causes the expansion of the whole He-buffer and of the overlying H-rich layer. As a consequence, the accreted WD overfills its own Roche lobe so that a large part (in some cases all) the previously accreted matter is lost from the binary system. As a matter of fact, the efficiency of the WD mass growth is very low and, hence, the number of SD systems suitable to produce a SN Ia is very low as compared to the observed explosion frequency in different galaxies. In some cases, the He-buffer piled-up via H-burning becomes so massive that the resulting He-flash is very strong and produces a detonation. In this case an explosion of SN Ia proportion is obtained, but the produced nucleosynthesis as well as the luminosity of the objects are in disagreement with those observed typical SNe Ia.
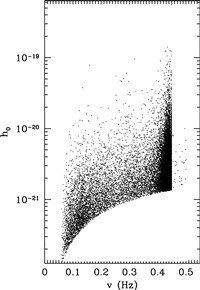
In DD systems it is assumed that the two CO WDs comes into contact due to gravitational wave radiation emission. When the less massive component, which is also the more expanded, first overfills its own Roche lobe, a dynamical mass transfer occurs. As a consequence, the WD completely disrupts, forming an accretion disk around the more massive companion. In this way CO rich matter is directly accreted, thus avoiding all the problems related to nuclear burning and related flash episodes occurring in the SD scenario. However, as it is well known, in merging systems the mass transfer rate is very high so that the accreting WD experiences an off-center ignition of carbon, thus producing an ONeMg WD, not a SN Ia event. In recent years, we suggested [5,6] that rotation plays a pivotal role in determining the evolution of DD systems. In fact, in these systems very high rotation velocity are attained so that rotation itself regulates the mass transfer rate, thus avoiding the off-center ignition of carbon and allowing the WD to increase its total mass up and above the canonical non-rotating Chandrasekhar mass limit (Rotating DD scenario). We show that, due to the continuous deposition of angular momentum, the accreting WD deforms into a Jacobi ellipsoid, thus emitting gravitational wave radiation (GWR) that stabilize the mass transfer rate. We also demonstrate that after all the matter in the accretion disk has been transferred to the WD, the explosion does not occur immediately, as the rapid rotation of the star prevents any contraction. In this way the explosion itself can occur only after several megayears, when, due to some physical processes, the accreted WD loses part of its angular momentum and experiences an homologous compression. This implies that it is practically impossible to detect the imprint of the progenitor into archive frames of a zone where a SN Ia explodes. As GWR emission is the main characteristic of the Rotating DD scenario, we determined the expected signal coming from galactic sources and we found that it could be easily detected with the next generation of space interferometers [7]. It is worth noticing that rotation could affect the evolution of both DD and SD systems, as well as that of binaries envisioned in the Core Degenerate scenario [8]. Hence, if rotation drive the evolution of CO WDs accreting matter, the imprint of the progenitor systems can be detected, independently of the adopted scenario for the progenitors. By analyzing the evolution of accreted CO WDs with total mass larger than MCh up to the explosion epoch, we found that during the last few month before explosion a strong emission in the soft-X band (0.5-2 keV) is associated with these objects. Such a feature can be used to confirm that the evolution up to the explosion is driven by rotation and, if so, to discriminate between different scenarios for the progenitors [9].
Recently, it has been suggested that the “real” progenitor systems of SNe Ia are CO WDs with a He-rich donor, such as a He star or a He WD. In this case, due to the continuous mass deposition, the CO WD increase its mass over about 0.9 Mʘ and, successively, a He-rich buffer massive enough is accumulated to cause the explosion of the star for He-detonation. We demonstrated that this evolutionary channel could produce explosive events similar to a typical SN Ia, even if their expected rate is too low to explain the observed SNe Ia rate [10,11].
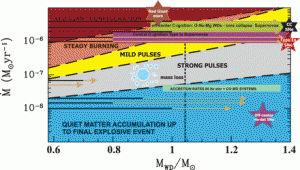
We also analyze the evolutionary properties of AM Canis Venaticorum (AM CV) systems by computing, for the first time, fully self-consistent models of mass transfer driven by GWR emission from a He WD to a CO WD considering at the same time the evolution of both the donor and the accretor. Our results show that explosive events for He-detonation as well as less luminous phenomena such as SNe .Ia are much less probable than previously estimated [12].
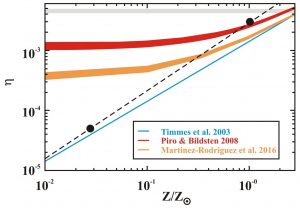
In collaboration with our colleagues from the UPC (Barcelona, Spain), the UGR (Granada Spain) and the TUD (Darmstadt, Germany) we analyzed the effects of the current uncertainties in the 12C+12C cross section on the observational properties of SNe Ia [13,14]. Moreover, we computed self-consistent models of the SNe Ia simmering phase, by solving simultaneously the equation describing the stellar structure and the evolution of the chemicals as determined by both nuclear burning and convection. By carefully treating the URCA processes, we were able to reconcile the neutronization level derived from abundance measurements of Cr and Mn in galactic supernova remnants with the average metallicity of the parent stellar population [15]. In particular, we demonstrated that the neutronization produced during the simmering phase strongly depends on the initial metallicity of the WD progenitors.
References:
- [1] Cassisi S., Iben, I.Jr, Tornambé A., 1998, ApJ, 496, 376
- [2] Piersanti L., Cassisi S., Iben I.Jr, Tornambé A., 1999, ApJ, 521, L59
- [3] Piersanti L., Cassisi S., Iben I.Jr, Tornambé A., 2000, ApJ, 535, 932
- [4] Piersanti L., Cassisi S., Iben I.Jr, Tornambé A., 2002, AIP Conference Proceedings, 637, 99
- [5] Piersanti L., Gagliardi S., Iben, I.Jr, Tornambé A., 2003, ApJ, 583, 885
- [6] Piersanti L., Gagliardi S., Iben, I.Jr, Tornambé A., 2003, ApJ, 598, 1229
- [7] Tornambé A., Piersanti L., 2013, MNRAS, 431, 1812
- [8] Ilkov M., Soker N., 2012, MNRAS, 419, 1695
- [9] Tornambé A., Piersanti L., Raimondo G., Del Grande R., 2017, to be submitted to MNRAS
- [10] Piersanti L., Tornambé A., Yungelson L., Straniero O., 2013, IAUS, 281, 209
- [11] Piersanti L., Tornambé A., Yungelson L., 2014, MNRAS, 445, 3239
- [12] Piersanti L., Tornambé A., Yungelson L., 2015, MNRAS, 452, 2897
- [13] Domìnguez I., Piersanti L., Bravo E., Tornambé A., Straniero O., Gagliardi S., 2006, ApJ, 644, 21
- [14] Bravo E., Domìnguez I., Badenes C., Piersanti L., Straniero O., 2010, ApJ, 711, L66
- [15] Piersanti L., Bravo E., Cristallo S., Domínguez I., Straniero O., Tornambé A., Martínez-Pinedo G., ApJ, 836, L9